Kárbọ̀nù
Àyọkà yìí únfẹ́ ìyílédèdà sí Yorùbá. Ẹ ran Wikipedia lọ́wọ́ ṣàtúnṣe sí ìyílédèdà |
![]() Graphite (left) and diamond (right), two allotropes of carbon | ||||||||||||||||||||||||||||||
Kárbọ̀nù | ||||||||||||||||||||||||||||||
---|---|---|---|---|---|---|---|---|---|---|---|---|---|---|---|---|---|---|---|---|---|---|---|---|---|---|---|---|---|---|
Ìhànsójú | clear (diamond) & black (graphite) | |||||||||||||||||||||||||||||
Ìwúwo átọ̀mù Ar, std(C) | [12.0096, 12.0116] conventional: 12.011 | |||||||||||||||||||||||||||||
Kárbọ̀nù ní orí tábìlì àyè | ||||||||||||||||||||||||||||||
| ||||||||||||||||||||||||||||||
Nọ́mbà átọ̀mù (Z) | 6 | |||||||||||||||||||||||||||||
Ẹgbẹ́ | group 14 (carbon group) | |||||||||||||||||||||||||||||
Àyè | àyè 2 | |||||||||||||||||||||||||||||
Àdìpọ̀ | Àdìpọ̀-p | |||||||||||||||||||||||||||||
Ẹ̀ka ẹ́límẹ́ntì | Reactive nonmetal | |||||||||||||||||||||||||||||
Ìtò ẹ̀lẹ́ktrọ́nù | [He] 2s2 2p2 | |||||||||||||||||||||||||||||
Iye ẹ̀lẹ́ktrọ́nù lórí ìpele kọ̀ọ̀kan | 2, 4 | |||||||||||||||||||||||||||||
Àwọn ohun ìní ara | ||||||||||||||||||||||||||||||
Ìfarahàn at STP | solid | |||||||||||||||||||||||||||||
Sublimation point | 3915 K (3642 °C, 6588 °F) | |||||||||||||||||||||||||||||
Kíki (near r.t.) | amorphous:[1] 1.8–2.1 g/cm3 diamond: 3.515 g/cm3 graphite: 2.267 g/cm3 | |||||||||||||||||||||||||||||
Triple point | 4600 K, 10800[2][3] kPa | |||||||||||||||||||||||||||||
Heat of fusion | 117 (graphite) kJ/mol | |||||||||||||||||||||||||||||
Molar heat capacity | 6.155 (diamond) 8.517 (graphite) J/(mol·K) | |||||||||||||||||||||||||||||
Atomic properties | ||||||||||||||||||||||||||||||
Oxidation states | −4, −3, −2, −1, 0, +1,[4] +2, +3,[5] +4[6] Àdàkọ:Infobox element/symbol-to-oxidation-state/comment | |||||||||||||||||||||||||||||
Electronegativity | Pauling scale: 2.55 | |||||||||||||||||||||||||||||
energies |
| |||||||||||||||||||||||||||||
Covalent radius | 77(sp³), 73(sp²), 69(sp) pm | |||||||||||||||||||||||||||||
Van der Waals radius | 170 pm | |||||||||||||||||||||||||||||
![]() | ||||||||||||||||||||||||||||||
Other properties | ||||||||||||||||||||||||||||||
Natural occurrence | primordial | |||||||||||||||||||||||||||||
Crystal structure | (diamond, clear) | |||||||||||||||||||||||||||||
Speed of sound thin rod | 18350 (diamond) m/s (at 20 °C) | |||||||||||||||||||||||||||||
Thermal expansion | 0.8 (diamond)[7] µm/(m·K) (at 25 °C) | |||||||||||||||||||||||||||||
Thermal conductivity | 900-2300 (diamond) 119-165 (graphite) W/(m·K) | |||||||||||||||||||||||||||||
Magnetic ordering | diamagnetic[8] | |||||||||||||||||||||||||||||
Young's modulus | 1050 (diamond)[7] GPa | |||||||||||||||||||||||||||||
Shear modulus | 478 (diamond)[7] GPa | |||||||||||||||||||||||||||||
Bulk modulus | 442 (diamond)[7] GPa | |||||||||||||||||||||||||||||
Poisson ratio | 0.1 (diamond)[7] | |||||||||||||||||||||||||||||
Mohs hardness | 10 (diamond) 1-2 (graphite) | |||||||||||||||||||||||||||||
CAS Number | 7440-44-0 | |||||||||||||||||||||||||||||
History | ||||||||||||||||||||||||||||||
Discovery | Egyptians and Sumerians[9] (3750 BC) | |||||||||||||||||||||||||||||
Recognized as an element by | Antoine Lavoisier[10] (1789) | |||||||||||||||||||||||||||||
Main isotopes of kárbọ̀nù | ||||||||||||||||||||||||||||||
| ||||||||||||||||||||||||||||||
Kárbọ̀nù (láti Látìnì: carbo "èédú") je ipilese kemika to ni ami-idamo C ati nomba atomu 6. Gẹ́gẹ́ bí ìkan nínú ẹgbẹ́ 14 lórí tábìlì ìdásìkò àwọn ẹ́límẹ̀ntì, ó jẹ́ aláìjẹ́-mẹ́tàlì àti afagbáramẹ́rindìmú—èyí jẹ́ pé ẹ̀lẹ́ktrọ́nù mẹ́rin wà láti dá ìsopọ̀ kẹ́míkà àjọfagbáradìmú. Àwọn ísótòpù kárbọ̀nù mẹ́ta ni wọ́n wà fún ra wọn, 12C àti 13C jẹ́ dídúró, nígbàtí 14C jẹ́ onítítànyindin, tóúntúká díẹ̀díẹ̀ pẹ̀lú àsìkò àbọ̀-ìgbéayé tó tó bíi ọdún 5,730.[14] Kárbọ̀nù ni ìkan nínú àwọn ẹ́límẹ̀ntì díẹ̀ tí wọ́n ti jẹ́ dídámọ̀ láti ìgbà àtijọ́.[15][16]
Orísi àwọn álótrópù kárbọ̀nù lọ́wà tí èyí tó gbajúmọ̀ nínú wọn jẹ́ gráfáìt, díámọ́ndì, àti kárbọ̀nù àìlétòara.[17] Àwọn ìní oníṣeẹ̀dá kárbọ̀nù yàtọ̀ gẹ́gẹ́bí irú álótrópù bá ṣe rí. Fún àpẹrẹ, díámọ́ndì jẹ́ híhannú, nígbàtí gráfáìt jẹ́ dídinú tó sì dúdú. Díámọ́ndì jẹ́ ìkan nínú àwọn èròjà tó le jùlọ, nígbàtí gráfáìt jẹ́ dídẹra dáadáa tó bẹ̀ẹ̀ láti fi kọ ìwé. Díámọ́ndì ní ìgbéwọra oníṣetanná tó kéré gidi, nígbàtí gráfáìt jẹ́ olùgbéwọra tó dára gidigidi. Lábẹ́ àwọn ìṣẹ̀lẹ̀ onídéédé, díámọ́ndì, nánótúbù kárbọ̀nù àti grafínnì ní ìgbéwọra onígbígbóná tó gajùlọ ní àrin àwọn èròjà tí a mọ̀.
Gbogbo àwọn álótrópù kárbọ̀nù jẹ́ aláralíle ní abẹ́ ìṣẹ̀lẹ̀ onídéédé tí gráfáìt jẹ́ irú èyí tó jẹ́ dídúró jùlọ níagbára onítigbígbóná. Wọ́n jẹ́ olódì sí kẹ́míkà bẹ́èsìni wọ́n fẹ́ ìgbónásí gíga láti túndaramọ́ra gan mọ́ ọ́ksíjìn. The most common Ipòàyè ìsọdiọ̀ksídì kárbọ̀nù nínú àwọn àdàpọ̀ aláìníọ́rgánì ni +4, nígbàtí +2 wà nínú mónọ́ksídì kárbọ̀nù àti àwọn metali irekoja karbonili miran. Orisun totobijulo karbonu alainiorgani ni okuta-efun, dolomaiti ati dioksidi karbonu, sugbon opo re tun wa ninu awon ìwalẹ̀ oniorgani èédú, peat, epo petroliomu ati klatarati methani. Karbonu se Carbon forms more adapo ju awon elimenti yiowu lo, bi milionu mewa adapo oniorgani ogidi lo wa titi doni, sibe awon yi ko ju ida die lo ninu gbogbo iye iru adapo karbonu to se e se labe isele onideede.[18]
Karbonu ni elimenti 15k to gbalejulo ni inu iwule Aye, ati elimenti kerin to gbale julo ninu agbala-aye gegebi iye akojo leyin háídrójìn, hílíọ̀mù, ati ọ́ksíjìn. O wa ninu gbogbo iru eda ohun alaye, be sini ninu ara eniyan karbonu ni elimenti keji to gbalejulo gegebi iye akojo (bi 18.5%) leyin oksijin.[19] Igbale yi, lapapo mo ipinsorisirisi awon adapo organiki ati agbara won lati da polimeri ni ibi igbonasi to wopo ni ile Aye, so elimenti yi di ipile kemika gbogbo ohun elemi.
Àwọn ìdámọ̀
[àtúnṣe | àtúnṣe àmìọ̀rọ̀]
Àwọn orísi ìdá tàbí álótrópù kárbọ̀nù (ẹ wo ìsàlẹ̀) ní nínú wọn ohun tó le jùlọ ní ìdáyé, díámọ̀ndì, àti èyi tó jẹ́ ìkan nínú ohun rírọ̀ jùlọ, gráfáìtì. Bákannáà, ó ní ìfẹ́ fún ìsopọ̀ mọ́ àwọn átọ̀mù kékeré míràn, bíi àwọn átọ̀mù kárbọ̀nù míràn, ó sí le dá ọ̀pọ̀ ìsopọ̀ àjọfagbáradìmú afẹsẹ̀múlẹ̀ mọ́ àwọn átọ̀mú báyìí. Nítorí ẹ̀yí, kárbọ̀nù le dá orísirísi àdàpọ̀ tó pọ̀ tó mílíọ́mù mẹ́ẹ̀wá; ogunlọ́gọ̀ gbogbo àwọn àdápọ̀ kẹ́míkà.[18] Carbon also has the highest sublimation point of all elements. At atmospheric pressure it has no melting point as its triple point is at 10.8 ± 0.2 MPa and 4,600 ± 300 K (~4,330 °C or 7,820 °F),[2][3] so it sublimates at about 3,900 K.[20][21]
Carbon sublimes in a carbon arc which has a temperature of about 5,800 K (5,530 °C; 9,980 °F). Thus, irrespective of its allotropic form, carbon remains solid at higher temperatures than the highest melting point metals such as tungsten or rhenium. Although thermodynamically prone to oxidation, carbon resists oxidation more effectively than elements such as iron and copper that are weaker reducing agents at room temperature.
Carbon compounds form the basis of all known life on Earth, and the carbon-nitrogen cycle provides some of the energy produced by the Sun and other stars. Although it forms an extraordinary variety of compounds, most forms of carbon are comparatively unreactive under normal conditions. At standard temperature and pressure, it resists all but the strongest oxidizers. It does not react with sulfuric acid, hydrochloric acid, chlorine or any alkalis. At elevated temperatures carbon reacts with oxygen to form carbon oxides, and will reduce such metal oxides as iron oxide to the metal. This exothermic reaction is used in the iron and steel industry to control the carbon content of steel:
- Fe3O4 + 4 C(s) → 3 Fe(s) + 4 CO(g)
with sulfur to form carbon disulfide and with steam in the coal-gas reaction:
- C(s) + H2O(g) → CO(g) + H2(g).
Carbon combines with some metals at high temperatures to form metallic carbides, such as the iron carbide cementite in steel, and tungsten carbide, widely used as an abrasive and for making hard tips for cutting tools.
As of 2009, graphene appears to be the strongest material ever tested.[22] However, the process of separating it from graphite will require some technological development before it is economical enough to be used in industrial processes.[23]
The system of carbon allotropes spans a range of extremes:
Synthetic nanocrystalline diamond is the hardest material known.[24] | Graphite is one of the softest materials known. |
Diamond is the ultimate abrasive. | Graphite is a very good lubricant, displaying superlubricity.[25] |
Diamond is an excellent electrical insulator.[26] | Graphite is a conductor of electricity.[27] |
Diamond is the best known naturally occurring thermal conductor | Some forms of graphite are used for thermal insulation (i.e. firebreaks and heat shields) |
Diamond is highly transparent. | Graphite is opaque. |
Diamond crystallizes in the cubic system. | Graphite crystallizes in the hexagonal system.[28] |
Amorphous carbon is completely isotropic. | Carbon nanotubes are among the most anisotropic materials ever produced. |
Allotropes
[àtúnṣe | àtúnṣe àmìọ̀rọ̀]Atomic carbon is a very short-lived species and, therefore, carbon is stabilized in various multi-atomic structures with different molecular configurations called allotropes. The three relatively well-known allotropes of carbon are amorphous carbon, graphite, and diamond. Once considered exotic, fullerenes are nowadays commonly synthesized and used in research; they include buckyballs,[29][30] carbon nanotubes,[31] carbon nanobuds[32] and nanofibers.[33][34] Several other exotic allotropes have also been discovered, such as lonsdaleite,[35] glassy carbon,[36] carbon nanofoam[37] and linear acetylenic carbon (carbyne).[38]
The amorphous form is an assortment of carbon atoms in a non-crystalline, irregular, glassy state, which is essentially graphite but not held in a crystalline macrostructure. It is present as a powder, and is the main constituent of substances such as charcoal, lampblack (soot) and activated carbon. At normal pressures carbon takes the form of graphite, in which each atom is bonded trigonally to three others in a plane composed of fused hexagonal rings, just like those in aromatic hydrocarbons.[39] The resulting network is 2-dimensional, and the resulting flat sheets are stacked and loosely bonded through weak van der Waals forces. This gives graphite its softness and its cleaving properties (the sheets slip easily past one another). Because of the delocalization of one of the outer electrons of each atom to form a π-cloud, graphite conducts electricity, but only in the plane of each covalently bonded sheet. This results in a lower bulk electrical conductivity for carbon than for most metals. The delocalization also accounts for the energetic stability of graphite over diamond at room temperature.
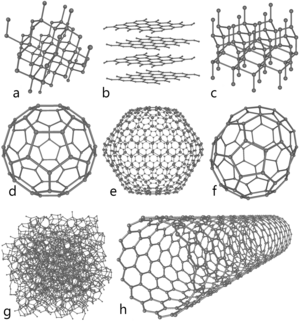
At very high pressures carbon forms the more compact allotrope diamond, having nearly twice the density of graphite. Here, each atom is bonded tetrahedrally to four others, thus making a 3-dimensional network of puckered six-membered rings of atoms. Diamond has the same cubic structure as silicon and germanium and because of the strength of the carbon-carbon bonds, it is the hardest naturally occurring substance in terms of resistance to scratching. Contrary to the popular belief that "diamonds are forever", they are in fact thermodynamically unstable under normal conditions and transform into graphite.[17] However, due to a high activation energy barrier, the transition into graphite is so extremely slow at room temperature as to be unnoticeable. Under some conditions, carbon crystallizes as lonsdaleite. This form has a hexagonal crystal lattice where all atoms are covalently bonded. Therefore, all properties of lonsdaleite are close to those of diamond.[35]
Fullerenes have a graphite-like structure, but instead of purely hexagonal packing, they also contain pentagons (or even heptagons) of carbon atoms, which bend the sheet into spheres, ellipses or cylinders. The properties of fullerenes (split into buckyballs, buckytubes and nanobuds) have not yet been fully analyzed and represent an intense area of research in nanomaterials. The names "fullerene" and "buckyball" are given after Richard Buckminster Fuller, popularizer of geodesic domes, which resemble the structure of fullerenes. The buckyballs are fairly large molecules formed completely of carbon bonded trigonally, forming spheroids (the best-known and simplest is the soccerball-shaped C60 buckminsterfullerene).[29] Carbon nanotubes are structurally similar to buckyballs, except that each atom is bonded trigonally in a curved sheet that forms a hollow cylinder.[30][31] Nanobuds were first reported in 2007 and are hybrid bucky tube/buckyball materials (buckyballs are covalently bonded to the outer wall of a nanotube) that combine the properties of both in a single structure.[32]
Of the other discovered allotropes, carbon nanofoam is a ferromagnetic allotrope discovered in 1997. It consists of a low-density cluster-assembly of carbon atoms strung together in a loose three-dimensional web, in which the atoms are bonded trigonally in six- and seven-membered rings. It is among the lightest known solids, with a density of about 2 kg/m3.[40] Similarly, glassy carbon contains a high proportion of closed porosity,[36] but contrary to normal graphite, the graphitic layers are not stacked like pages in a book, but have a more random arrangement. Linear acetylenic carbon[38] has the chemical structure[38] -(C:::C)n-. Carbon in this modification is linear with sp orbital hybridization, and is a polymer with alternating single and triple bonds. This type of carbyne is of considerable interest to nanotechnology as its Young's modulus is forty times that of the hardest known material – diamond.[41]
Occurrence
[àtúnṣe | àtúnṣe àmìọ̀rọ̀]


Carbon is the fourth most abundant chemical element in the universe by mass after hydrogen, helium, and oxygen. Carbon is abundant in the Sun, stars, comets, and in the atmospheres of most planets. Some meteorites contain microscopic diamonds that were formed when the solar system was still a protoplanetary disk. Microscopic diamonds may also be formed by the intense pressure and high temperature at the sites of meteorite impacts.[42]
In combination with oxygen in carbon dioxide, carbon is found in the Earth's atmosphere (approximately 810 gigatonnes of carbon) and dissolved in all water bodies (approximately 36,000 gigatonnes of carbon). Around 1,900 gigatonnes of carbon are present in the biosphere. Hydrocarbons (such as coal, petroleum, and natural gas) contain carbon as well—coal "reserves" (not "resources") amount to around 900 gigatonnes, and oil reserves around 150 gigatonnes. Proven sources of natural gas are about 175 1012 cubic metres (representing about 105 gigatonnes carbon), but it is estimated that there are also about 900 1012 cubic metres of "unconventional" gas such as shale gas, representing about 540 gigatonnes of carbon.[43] (In the past, quantities of hydrocarbons were greater. In the period from 1751 to 2008 about 347 gigatonnes of carbon were released as carbon dioxide to the atmosphere from burning of fossil fuels.[44]) Carbon is also locked up as methane and methane hydrates in polar regions. It is estimated that at least 1,400 Gt of carbon is in this form just in (and under) the submarine permafrost of the Siberian Shelf.[45]
Carbon is a major component in very large masses of carbonate rock (limestone, dolomite, marble and so on). Coal is the largest commercial source of mineral carbon, accounting for 4,000 gigatonnes or 80% of fossil carbon fuel.[46] It is also rich in carbon – for example, anthracite contains 92–98%.[47]
As for individual carbon allotropes, graphite is found in large quantities in the United States (mostly in New York and Texas), Russia, Mexico, Greenland, and India. Natural diamonds occur in the rock kimberlite, found in ancient volcanic "necks", or "pipes". Most diamond deposits are in Africa, notably in South Africa, Namibia, Botswana, the Republic of the Congo, and Sierra Leone. There are also deposits in Arkansas, Canada, the Russian Arctic, Brazil and in Northern and Western Australia. Diamonds are now also being recovered from the ocean floor off the Cape of Good Hope. However, though diamonds are found naturally, about 30% of all industrial diamonds used in the U.S. are now made synthetically.
Carbon-14 is formed in upper layers of the troposphere and the stratosphere, at altitudes of 9–15 km, by a reaction that is precipitated by cosmic rays.[48] Thermal neutrons are produced that collide with the nuclei of nitrogen-14, forming carbon-14 and a proton.
Isotopes
[àtúnṣe | àtúnṣe àmìọ̀rọ̀]Isotopes of carbon are atomic nuclei that contain six protons plus a number of neutrons (varying from 2 to 16). Carbon has two stable, naturally occurring isotopes.[14] The isotope carbon-12 (12C) forms 98.93% of the carbon on Earth, while carbon-13 (13C) forms the remaining 1.07%.[14] The concentration of 12C is further increased in biological materials because biochemical reactions discriminate against 13C.[49] In 1961, the International Union of Pure and Applied Chemistry (IUPAC) adopted the isotope carbon-12 as the basis for atomic weights.[50] Identification of carbon in NMR experiments is done with the isotope 13C.
Carbon-14 (14C) is a naturally occurring radioisotope which occurs in trace amounts on Earth of up to 1 part per trillion (0.0000000001%), mostly confined to the atmosphere and superficial deposits, particularly of peat and other organic materials.[51] This isotope decays by 0.158 MeV β− emission. Because of its relatively short half-life of 5730 years, 14C is virtually absent in ancient rocks, but is created in the upper atmosphere (lower stratosphere and upper troposphere) by interaction of nitrogen with cosmic rays.[52] The abundance of 14C in the atmosphere and in living organisms is almost constant, but decreases predictably in their bodies after death. This principle is used in radiocarbon dating, invented in 1949, which has been used extensively to determine the age of carbonaceous materials with ages up to about 40,000 years.[53][54]
There are 15 known isotopes of carbon and the shortest-lived of these is 8C which decays through proton emission and alpha decay and has a half-life of 1.98739x10−21 s.[55] The exotic 19C exhibits a nuclear halo, which means its radius is appreciably larger than would be expected if the nucleus were a sphere of constant density.[56]
Formation in stars
[àtúnṣe | àtúnṣe àmìọ̀rọ̀]Formation of the carbon atomic nucleus requires a nearly simultaneous triple collision of alpha particles (helium nuclei) within the core of a giant or supergiant star which is known as the triple-alpha process, as the products of further nuclear fusion reactions of helium with hydrogen or another helium nucleus produce lithium-5 and beryllium-8 respectively, both of which are highly unstable and decay almost instantly back into smaller nuclei.[57] This happens in conditions of temperatures over 100 megakelvin and helium concentration that the rapid expansion and cooling of the early universe prohibited, and therefore no significant carbon was created during the Big Bang. Instead, the interiors of stars in the horizontal branch transform three helium nuclei into carbon by means of this triple-alpha process.[58] In order to be available for formation of life as we know it, this carbon must then later be scattered into space as dust, in supernova explosions, as part of the material which later forms second, third-generation star systems which have planets accreted from such dust.[59] The Solar System is one such third-generation star system. Another of the fusion mechanisms powering stars is the CNO cycle, in which carbon acts as a catalyst to allow the reaction to proceed.
Rotational transitions of various isotopic forms of carbon monoxide (for example, 12CO, 13CO, and C18O) are detectable in the submillimeter wavelength range, and are used in the study of newly forming stars in molecular clouds.[60]
Carbon cycle
[àtúnṣe | àtúnṣe àmìọ̀rọ̀]
Under terrestrial conditions, conversion of one element to another is very rare. Therefore, the amount of carbon on Earth is effectively constant. Thus, processes that use carbon must obtain it somewhere and dispose of it somewhere else. The paths that carbon follows in the environment make up the carbon cycle. For example, plants draw carbon dioxide out of their environment and use it to build biomass, as in carbon respiration or the Calvin cycle, a process of carbon fixation. Some of this biomass is eaten by animals, whereas some carbon is exhaled by animals as carbon dioxide. The carbon cycle is considerably more complicated than this short loop; for example, some carbon dioxide is dissolved in the oceans; dead plant or animal matter may become petroleum or coal, which can burn with the release of carbon, should bacteria not consume it.[61][62]
![]() |
Àyọkà yìí tàbí apá rẹ̀ únfẹ́ àtúnṣe sí. Ẹ le fẹ̀ jù báyìí lọ tàbí kí ẹ ṣàtúnṣe rẹ̀ lọ́nà tí yíò mu kúnrẹ́rẹ́. Ẹ ran Wikipedia lọ́wọ́ láti fẹ̀ẹ́ jù báyìí lọ. |
Itokasi
[àtúnṣe | àtúnṣe àmìọ̀rọ̀]- ↑ Àdàkọ:RubberBible86th
- ↑ 2.0 2.1 Haaland, D (1976). "Graphite-liquid-vapor triple point pressure and the density of liquid carbon". Carbon 14 (6): 357. doi:10.1016/0008-6223(76)90010-5.
- ↑ 3.0 3.1 Savvatimskiy, A (2005). "Measurements of the melting point of graphite and the properties of liquid carbon (a review for 1963–2003)". Carbon 43 (6): 1115. doi:10.1016/j.carbon.2004.12.027.
- ↑ "Fourier Transform Spectroscopy of the Electronic Transition of the Jet-Cooled CCI Free Radical" (PDF). Retrieved 2007-12-06.
- ↑ "Fourier Transform Spectroscopy of the System of CP" (PDF). Retrieved 2007-12-06.
- ↑ "Carbon: Binary compounds". Retrieved 2007-12-06.
- ↑ 7.0 7.1 7.2 7.3 7.4 Properties of diamond, Ioffe Institute Database
- ↑ Magnetic susceptibility of the elements and inorganic compounds, in Handbook of Chemistry and Physics 81st edition, CRC press.
- ↑ "History of Carbon and Carbon Materials - Center for Applied Energy Research - University of Kentucky". Caer.uky.edu. Retrieved 2008-09-12.
- ↑ Senese, Fred (200-09-09). "Who discovered carbon?". Frostburg State University. Retrieved 2007-11-24. Check date values in:
|date=
(help) - ↑ "Fourier Transform Spectroscopy of the System of CP" (PDF). Retrieved 2007-12-06.
- ↑ "Fourier Transform Spectroscopy of the Electronic Transition of the Jet-Cooled CCI Free Radical" (PDF). Retrieved 2007-12-06.
- ↑ "Carbon: Binary compounds". Retrieved 2007-12-06.
- ↑ 14.0 14.1 14.2 "Carbon – Naturally occurring isotopes". WebElements Periodic Table. Retrieved 2008-10-09.
- ↑ "Periodic Table: Date of Discovery". Chemical Elements.com. Retrieved 2007-03-13.
- ↑ "Timeline of Element Discovery". Retrieved 2007-03-13.[Ìjápọ̀ tí kò ṣiṣẹ́ mọ́]
- ↑ 17.0 17.1 "World of Carbon – Interactive Nano-visulisation in Science &Engineering Edukation (IN-VSEE)". Retrieved 2008-10-09.
- ↑ 18.0 18.1 Chemistry Operations (December 15, 2003). "Carbon". Los Alamos National Laboratory. Archived from the original on 2008-09-13. Retrieved 2008-10-09.
- ↑ "Biological Abundance of Elements". The Internet Encyclopedia of Science. Retrieved 2008-10-09.
- ↑ Greenville Whittaker, A. (1978). "The controversial carbon solid−liquid−vapour triple point". Nature 276 (5689): 695–696. Bibcode 1978Natur.276..695W. doi:10.1038/276695a0.
- ↑ Zazula, J. M. (1997). "On Graphite Transformations at High Temperature and Pressure Induced by Absorption of the LHC Beam". CERN. Archived from the original on 2009-03-25. https://web.archive.org/web/20090325230751/http://lbruno.home.cern.ch/lbruno/documents/Bibliography/LHC_Note_78.pdf. Retrieved 2009-06-06.
- ↑ Lee, C.; Wei, X.; Kysar, J. W.; Hone, J. (2008). "Measurement of the Elastic Properties and Intrinsic Strength of Monolayer Graphene". Science 321 (5887): 385–8. Bibcode 2008Sci...321..385L. doi:10.1126/science.1157996. PMID 18635798. Lay summary.[Ìjápọ̀ tí kò ṣiṣẹ́ mọ́]
- ↑ Sanderson, Bill (2008-08-25). "Toughest Stuff Known to Man : Discovery Opens Door to Space Elevator". nypost.com. Archived from the original on 2008-09-06. Retrieved 2008-10-09.
- ↑ Irifune, Tetsuo; Kurio, Ayako; Sakamoto, Shizue; Inoue, Toru; Sumiya, Hitoshi (2003). "Materials: Ultrahard polycrystalline diamond from graphite". Nature 421 (6923): 599–600. doi:10.1038/421599b. PMID 12571587.
- ↑ Dienwiebel, Martin; Verhoeven, Gertjan; Pradeep, Namboodiri; Frenken, Joost; Heimberg, Jennifer; Zandbergen, Henny (2004). "Superlubricity of Graphite". Physical Review Letters 92 (12). doi:10.1103/PhysRevLett.92.126101. http://www.physics.leidenuniv.nl/sections/cm/ip/group/PDF/Phys.rev.lett/2004/92(2004)12601.pdf.
- ↑ Collins, A.T. (1993). "The Optical and Electronic Properties of Semiconducting Diamond". Philosophical Transactions of the Royal Society A 342 (1664): 233–244. Bibcode 1993RSPTA.342..233C. doi:10.1098/rsta.1993.0017.
- ↑ Deprez, N.; McLachan, D. S. (1988). "The analysis of the electrical conductivity of graphite conductivity of graphite powders during compaction". Journal of Physics D: Applied Physics (Institute of Physics) 21 (1): 101. Bibcode 1988JPhD...21..101D. doi:10.1088/0022-3727/21/1/015.
- ↑ Delhaes, P. (2001). Graphite and Precursors. CRC Press. ISBN 90-5699-228-7. http://books.google.com/?id=7p2pgNOWPbEC&pg=PA146.
- ↑ 29.0 29.1 Àṣìṣe ìtọ́kasí: Invalid
<ref>
tag; no text was provided for refs namedbuckyballs
- ↑ 30.0 30.1 Ebbesen, T. W., ed (1997). Carbon nanotubes—preparation and properties. Boca Raton, Florida: CRC Press. ISBN 0-8493-9602-6.
- ↑ 31.0 31.1 Dresselhaus, M. S.; Dresselhaus, G.; Avouris, Ph., ed (2001). "Carbon nanotubes: synthesis, structures, properties and applications". Topics in Applied Physics (Berlin: Springer) 80. ISBN 3-540-41086-4.
- ↑ 32.0 32.1 Nasibulin, Albert G.; Pikhitsa, P. V.; Jiang, H.; Brown, D. P.; Krasheninnikov, A. V.; Anisimov, A. S.; Queipo, P.; Moisala, A. et al. (2007). "A novel hybrid carbon material". Nature Nanotechnology 2 (3): 156–161. Bibcode 2007NatNa...2..156N. doi:10.1038/nnano.2007.37. PMID 18654245.
- ↑ Nasibulin, A; Anisimov, Anton S.; Pikhitsa, Peter V.; Jiang, Hua; Brown, David P.; Choi, Mansoo; Kauppinen, Esko I. (2007). "Investigations of NanoBud formation". Chemical Physics Letters 446: 109–114. Bibcode 2007CPL...446..109N. doi:10.1016/j.cplett.2007.08.050.
- ↑ Vieira, R; Ledoux, Marc-Jacques; Pham-Huu, Cuong (2004). "Synthesis and characterisation of carbon nanofibers with macroscopic shaping formed by catalytic decomposition of C2H6/H2 over nickel catalyst". Applied Catalysis A 274: 1–8. doi:10.1016/j.apcata.2004.04.008.
- ↑ 35.0 35.1 Clifford, Frondel; Marvin, Ursula B. (1967). "Lonsdaleite, a new hexagonal polymorph of diamond". Nature 214 (5088): 587–589. Bibcode 1967Natur.214..587F. doi:10.1038/214587a0.
- ↑ 36.0 36.1 Àṣìṣe ìtọ́kasí: Invalid
<ref>
tag; no text was provided for refs namedglassy carbon
- ↑ Rode, A. V.; Hyde, S. T.; Gamaly, E. G.; Elliman, R. G.; McKenzie, D. R.; Bulcock, S. (1999). "Structural analysis of a carbon foam formed by high pulse-rate laser ablation". Applied Physics A-Materials Science & Processing 69 (7): S755–S758. doi:10.1007/s003390051522.
- ↑ 38.0 38.1 38.2 Heimann, Robert Bertram; Evsyukov, Sergey E. and Kavan, Ladislav (28 February 1999). Carbyne and carbynoid structures. Springer. pp. 1–. ISBN 978-0-7923-5323-2. http://books.google.com/books?id=swSQZcTmo_4C&pg=PA1. Retrieved 6 July 2011.
- ↑ Jenkins, Edgar (1973). The polymorphism of elements and compounds. Taylor & Francis. p. 30. ISBN 0-423-87500-0. http://books.google.com/books?id=XNYOAAAAQAAJ&pg=PA30. Retrieved 2011-05-01.
- ↑ Schewe, Phil and Stein, Ben (March 26, 2004). "Carbon Nanofoam is the World's First Pure Carbon Magnet". Physics News Update 678 (1). Archived from the original on March 7, 2012. https://web.archive.org/web/20120307104655/http://www.aip.org/pnu/2004/split/678-1.html. Retrieved December 9, 2012.
- ↑ Itzhaki, Lior; Altus, Eli; Basch, Harold; Hoz, Shmaryahu (2005). "Harder than Diamond: Determining the Cross-Sectional Area and Young's Modulus of Molecular Rods". Angew. Chem. Int. Ed. 44 (45): 7432–5. doi:10.1002/ange.200502448. PMID 16240306.
- ↑ Mark, Kathleen (1987). Meteorite Craters. University of Arizona Press. ISBN 0-8165-0902-6.
- ↑ "Wonderfuel: Welcome to the age of unconventional gas" by Helen Knight, New Scientist, 12 June 2010, pp. 44–7.
- ↑ Calculated from file global.1751_2008.csv in [1] from the Carbon Dioxide Information Analysis Center.
- ↑ N. Shakhova, I. Semiletov, A. Salyuk, D. Kosmach (2008), Anomalies of methane in the atmosphere over the East Siberian shelf: Is there any sign of methane leakage from shallow shelf hydrates? Archived 2012-12-22 at the Wayback Machine., EGU General Assembly 2008, Geophysical Research Abstracts, 10, EGU2008-A-01526
- ↑ Kasting, James (1998). "The Carbon Cycle, Climate, and the Long-Term Effects of Fossil Fuel Burning". Consequences: the Nature and Implication of Environmental Change 4 (1). http://gcrio.org/CONSEQUENCES/vol4no1/carbcycle.html.
- ↑ Stefanenko, R. (1983). Coal Mining Technology: Theory and Practice. Society for Mining Metallurgy. ISBN 0-89520-404-5.
- ↑ Carbon-14 formation
- ↑ Gannes, Leonard Z.; Del Rio, Carlos Martı́nez; Koch, Paul (1998). "Natural Abundance Variations in Stable Isotopes and their Potential Uses in Animal Physiological Ecology". Comparative Biochemistry and Physiology – Part A: Molecular & Integrative Physiology 119 (3): 725–737. doi:10.1016/S1095-6433(98)01016-2.
- ↑ "Official SI Unit definitions". Retrieved 2007-12-21.
- ↑ Brown, Tom (March 1, 2006). "Carbon Goes Full Circle in the Amazon". Lawrence Livermore National Laboratory. Retrieved 2007-11-25.
- ↑ Bowman, S. (1990). Interpreting the past: Radiocarbon dating. British Museum Press. ISBN 0-7141-2047-2.
- ↑ Libby, W. F. (1952). Radiocarbon dating. Chicago University Press and references therein.
- ↑ Westgren, A. (1960). "The Nobel Prize in Chemistry 1960". Nobel Foundation. Retrieved 2007-11-25.
- ↑ "Use query for carbon-8". barwinski.net. Retrieved 2007-12-21.
- ↑ Watson, A. (1999). "Beaming Into the Dark Corners of the Nuclear Kitchen". Science 286 (5437): 28–31. doi:10.1126/science.286.5437.28. http://www.sciencemag.org/cgi/content/full/286/5437/28?ck=nck.
- ↑ Audi, G; Bersillon, O.; Blachot, J.; Wapstra, A.H. (1997). "The Nubase evaluation of nuclear and decay properties". Nuclear Physics A 624: 1. Bibcode 1997NuPhA.624....1A. doi:10.1016/S0375-9474(97)00482-X. http://amdc.in2p3.fr/nubase/nubase97.pdf.
- ↑ Ostlie, D.A. and Carroll, B.W. (2007). An Introduction to Modern Stellar Astrophysics. Addison Wesley, San Francisco. ISBN 0-8053-0348-0.
- ↑ Whittet, D. C. B. (2003). Dust in the Galactic Environment. CRC Press. pp. 45–46. ISBN 0-7503-0624-6.
- ↑ Pikelʹner, Solomon Borisovich (1977). Star formation. Springer. pp. 38–. ISBN 978-90-277-0796-3. http://books.google.com/books?id=qbGLgcxnfpIC&pg=PA38. Retrieved 6 July 2011.
- ↑ Falkowski, P.; Scholes, RJ; Boyle, E; Canadell, J; Canfield, D; Elser, J; Gruber, N; Hibbard, K et al. (2000). "The Global Carbon Cycle: A Test of Our Knowledge of Earth as a System". Science 290 (5490): 291–296. Bibcode 2000Sci...290..291F. doi:10.1126/science.290.5490.291. PMID 11030643.
- ↑ Smith, T. M.; Cramer, W. P.; Dixon, R. K.; Leemans, R.; Neilson, R. P.; Solomon, A. M. (1993). "The global terrestrial carbon cycle". Water, Air, & Soil Pollution 70: 19–37. doi:10.1007/BF01104986.
Tábìlì ìdásìkò | |||||||||||||||||||||||||||||||||||||||||||
---|---|---|---|---|---|---|---|---|---|---|---|---|---|---|---|---|---|---|---|---|---|---|---|---|---|---|---|---|---|---|---|---|---|---|---|---|---|---|---|---|---|---|---|
1 | 2 | 3 | 4 | 5 | 6 | 7 | 8 | 9 | 10 | 11 | 12 | 13 | 14 | 15 | 16 | 17 | 18 | ||||||||||||||||||||||||||
1 | H | He | |||||||||||||||||||||||||||||||||||||||||
2 | Li | Be | B | C | N | O | F | Ne | |||||||||||||||||||||||||||||||||||
3 | Na | Mg | Al | Si | P | S | Cl | Ar | |||||||||||||||||||||||||||||||||||
4 | K | Ca | Sc | Ti | V | Cr | Mn | Fe | Co | Ni | Cu | Zn | Ga | Ge | As | Se | Br | Kr | |||||||||||||||||||||||||
5 | Rb | Sr | Y | Zr | Nb | Mo | Tc | Ru | Rh | Pd | Ag | Cd | In | Sn | Sb | Te | I | Xe | |||||||||||||||||||||||||
6 | Cs | Ba | La | Ce | Pr | Nd | Pm | Sm | Eu | Gd | Tb | Dy | Ho | Er | Tm | Yb | Lu | Hf | Ta | W | Re | Os | Ir | Pt | Au | Hg | Tl | Pb | Bi | Po | At | Rn | |||||||||||
7 | Fr | Ra | Ac | Th | Pa | U | Np | Pu | Am | Cm | Bk | Cf | Es | Fm | Md | No | Lr | Rf | Db | Sg | Bh | Hs | Mt | Ds | Rg | Cn | Nh | Fl | Mc | Lv | Ts | Og | |||||||||||
| |||||||||||||||||||||||||||||||||||||||||||
|
- Pages using duplicate arguments in template calls
- Pages with reference errors
- CS1 errors: dates
- All articles with dead external links
- Articles with dead external links from June 2012
- Articles with invalid date parameter in template
- Pages containing cite templates with deprecated parameters
- Webarchive template wayback links
- Pages using infobox element with unknown parameters
- Articles containing Látìnì-language text
- Kárbọ̀nù
- Àwọn ẹ́límẹ̀ntì kẹ́míkà
- Àwọn aláìjẹ́-mẹ́tàlì